Spectrometers: Resolution vs. Sensitivity
Spectrometry is a measurement method in which information about a specific spectrum of physical properties is obtained from a sample. These properties are separated and analyzed individually. An exact breakdown of the properties of this sample is created, which allows conclusions to be drawn about its composition. In the case of optical spectrometers, the physical property of this sample examined is the emission, absorption or reflection of light.
Light is a mixture of electromagnetic waves
From a physical point of view, light is a specific area of electromagnetic radiation. These are waves from coupled electrical and magnetic fields that propagate through space at a certain wavelength. So-called visible light is in a wavelength range from about 380 (violet) to 750 nm (red), whereby the longer the wavelength, the lower the energy. This wavelength range does not differ fundamentally from other wavelengths. The radiation from our sun is strongest at these frequencies, and therefore our eyes have developed in such a way that they can perceive precisely these wavelengths. Adjacent to this are ultraviolet rays (UV) and high infrared rays (IR) in the low wavelength range, which are outside of human eyesight. Other animals such as Insects, birds or some snakes can still visualize these rays just behind the edges of the visible light. People cannot see IR radiation, but they can perceive it as heat through the skin.
Light on Earth comes primarily from the sun. It emits a spectrum of electromagnetic radiation, the greatest intensity of which is in the range of visible light. This radiation moves through space at the speed of light and strikes a multitude of different substances on Earth. If these substances completely reflected the entire spectrum of sunlight, the entire world would be white for our eyes. In reality, however, this practically never happens. Instead, almost all substances absorb parts of the visible spectrum. Part of the light therefore no longer reaches our eyes - a color impression arises.
The entire color spectrum of white sunlight can be viewed clearly on a rainbow. It arises when the light is refracted and reflected in countless raindrops. The single beam of light enters the water plug and is broken at this barrier. This happens because light is refracted at the transition between substances of different densities (in this case water and air). The angle of refraction is somewhat dependent on the wavelength. As a result, the light is fanned out into its sub-frequencies - a rainbow is created.
But even without rain, the white light of the sun is scattered in the atmosphere. The smallest particles play an important role in this. Their composition, which is typical of the earth, primarily ensures that blue light components are scattered. This is how the sky, and ultimately the planet viewed from space, appears blue. Dyes also react very specifically to light, e.g. the chlorophyll in the leaves of plants. It absorbs blue and red light to generate energy for photosynthesis. The green parts in the light, however, are reflected. Therefore leaves appear green to us.
In this way, different materials or atomic structures react naturally to light in a very specific way. Conversely, the response to incident light can be examined to draw conclusions about the molecular structure or composition of a sample. This principle is also enabling optical spectroscopy.
An optical spectrometer examines the optical properties of a sample
Optical spectroscopy is a common method for examining solids. An optical spectrometer initially consists of a light source, the light of which is then refracted in a monochromator and only transmitted in a certain wavelength range. This defined light beam then runs through the sample to be examined and finally strikes a detector which can recognize to what extent the sample has changed the defined light beam. Prior to the actual measurement, the optical properties were of course carefully researched, so that a quick comparison of the measurement result with these findings allows precise conclusions to be drawn about the sample.
Resolution vs. Sensitivity in spectrometers
The exact structure of the detector determines the resolution and sensitivity of the spectrometer. Both are inversely proportional to each other. So if the design's resolution capacity is maximized, sensitivity suffers and vice versa.
Ultimately, the detector consists of a series of pixels, each of which responds to the light of a specific wavelength range. The best resolution is achieved by setting the individual areas to which a pixel responds very narrowly. This leads to a clear delimitation of individual wavelengths, but also ensures that only a few photons hit exactly this pixel. In order to still be able to detect weaker signals well, the measuring time must be extended. Very weak signals can often not be perceived because too few photons arrive. In order to either reduce the measurement time or to better detect the weak signals, the wavelength range per pixel can be chosen larger. This means that more photons can be detected in a shorter time. The sensitivity of the measuring device is therefore increased. However, this naturally leads to the boundaries between the areas becoming less clear and thus reducing the resolution.
Another aspect is the signal-to-noise ratio, which turns out to be more unfavorable at high resolution because fewer signal photons hit a pixel. This means that the more photons can hit a single pixel, because perhaps the wavelength range is chosen wider, the lower the background noise ratio. The noise can be greatly reduced by conducting multiple measuring cycles. This in turn has the consequence that measurements with spectrometers optimized for high resolution take longer overall.
You might also like
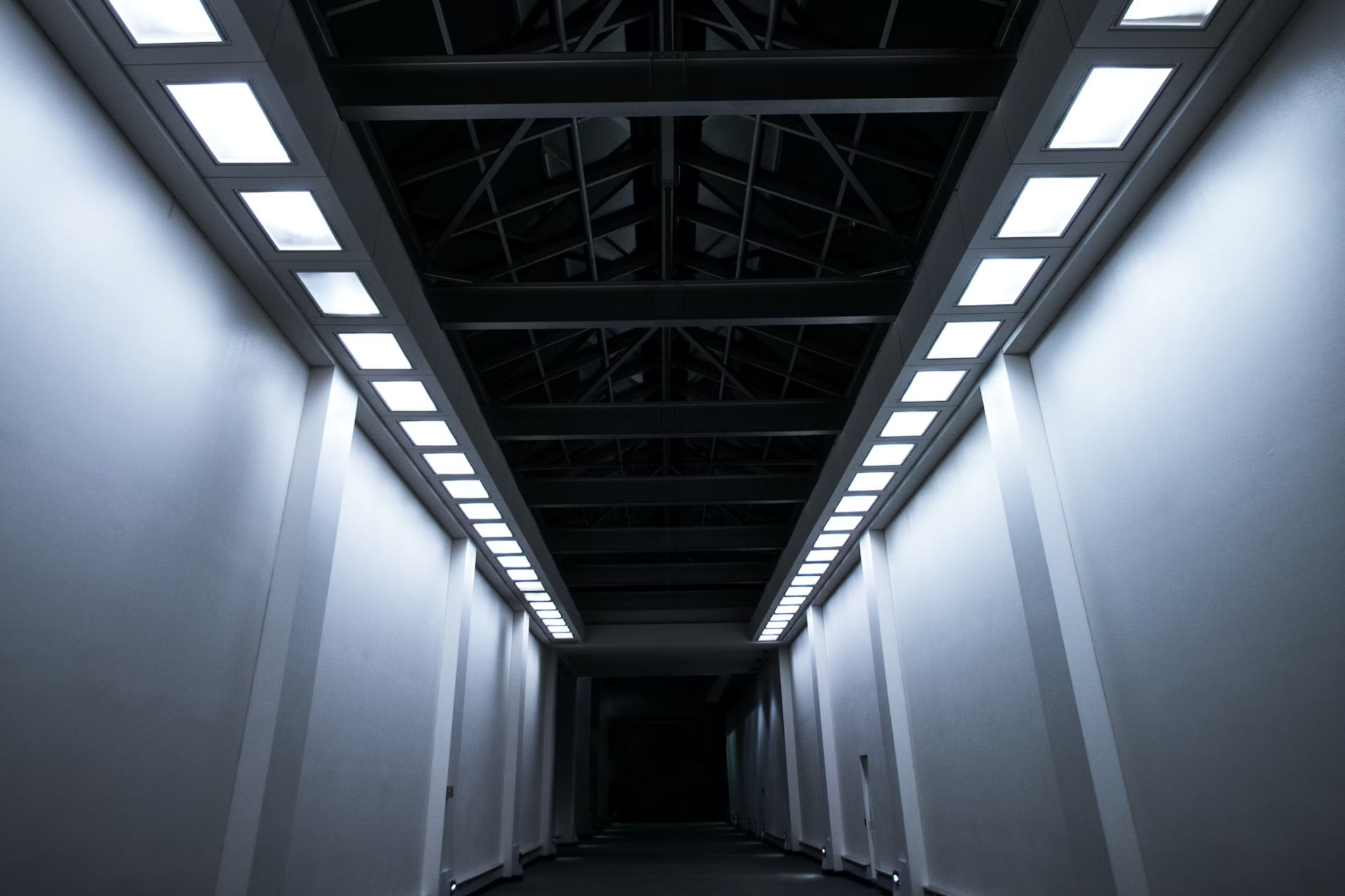
Nits, Lux, Lumen, Candela - calculating with light and lighting
Luminous flux, luminous intensity, luminance and co. - when is which quantity used and how can they be compared?