Spectral resolution explained
One of the important properties of a spectrometer is its spectral resolution. It states how finely different narrow wavelengths can be made visible as deflections or peaks.
An example: A spectrometer is sensitive to wavelengths from 420nm to 780nm. The measurable range is therefore 360nm wide. The spectral resolution is 12nm, which means 360/12 = 30 peaks could be detected simultaneously.
It is sometimes assumed that the number of sensor pixels determines the resolution. A spectrometer with a 256-pixel sensor line and a measuring range of 360nm would then have a spectral resolution of 360/256 = 1.4nm.
At first glance that makes sense, because the incident light is distributed over these 256 pixels, right? That's actually true, but at this point a lot has already happened to the light that travelled from the inlet to these sensor pixels.
In a spectrometer, three elements are mainly responsible for the effective spectral resolution: the slit, the diffraction grating and the sensor line already mentioned (in some designs the slit and / or diffraction grating are replaced by other components, though these serve the same purposes, respectively).
The narrower the entry slit, the finer the incoming signal can be. However, the gap cannot be chosen to be infinitely fine, because at some point the incoming radiation is so strongly bent that it is superimposed behind the gap and the result becomes blurred again.
Since the diffraction depends on the wavelength (explained here for the formation of a rainbow) there is no single optimal slit width - instead it depends on the measuring range for which the spectrometer was developed.
The same applies to the next stage of the incident radiation: the diffraction grating. The task of this element is to fan out the different, overlapping wavelengths in such a way that they irradiate the full width of the sensor line at consistent intervals.
Between the gap and the grid or the grid and the sensor line, there are also lenses that do not behave like perfect theoretical elements in practice, but may have slight deviations from the planned behavior due to impurities or minimal fluctuations in the manufacturing process. In practice, however, these days the design of the slit and grating is of far greater importance.
measuring spectral resolution
The expected spectral resolution can be calculated if the planned measuring range is known. In this way, manufacturers combine columns, grids and sensor lines to create a measuring instrument that fits the anticipated application scenario.
The spectral resolution achieved in practice can, however, also be determined empirically. For this purpose, light sources which are known to have very narrow-band peaks at a very specific wavelength are measured using the spectrometer. One common example are Mercury vapor lamps. The actual peak of the light source should be significantly narrower than the expected spectral resolution of the device.
If you now measure such a radiation source and look at the result, you will get a detected peak that is wider than the actual, known peak of the source.
This peak can now be measured. Spectral resolution is most commonly defined as FWHM - full width at half maximum. The width of the peak is measured, namely at the point where the measured peak has reached exactly half of the maximum deflection (see visualization).
Is a high spectral resolution always optimal?
No. As with many other measuring instruments, there is always a tradeoff between resolution and sensitivity with spectrometers. The higher the spectral resolution, the fewer photons arrive at a single pixel in the sensor array. If the number of photons falls below a certain limit, the signal can no longer be distinguished from the background noise - similar to a noisy cell phone photo of a night scene. So it all depends on the purpose: If it is clear that you have enough time and a strong signal for a measurement, then a spectrometer with a very fine spectral resolution can be selected. This is the typical scenario for laboratory spectrometers.
However, if many measurements have to be carried out within a second, for example when continuously measuring products passing through a production line, then sensitivity is of greater importance. This is the typical field of application for so-called process spectrometers or inline spectrometers. These devices are optimized to deliver a clear result in a split second.
You might also like
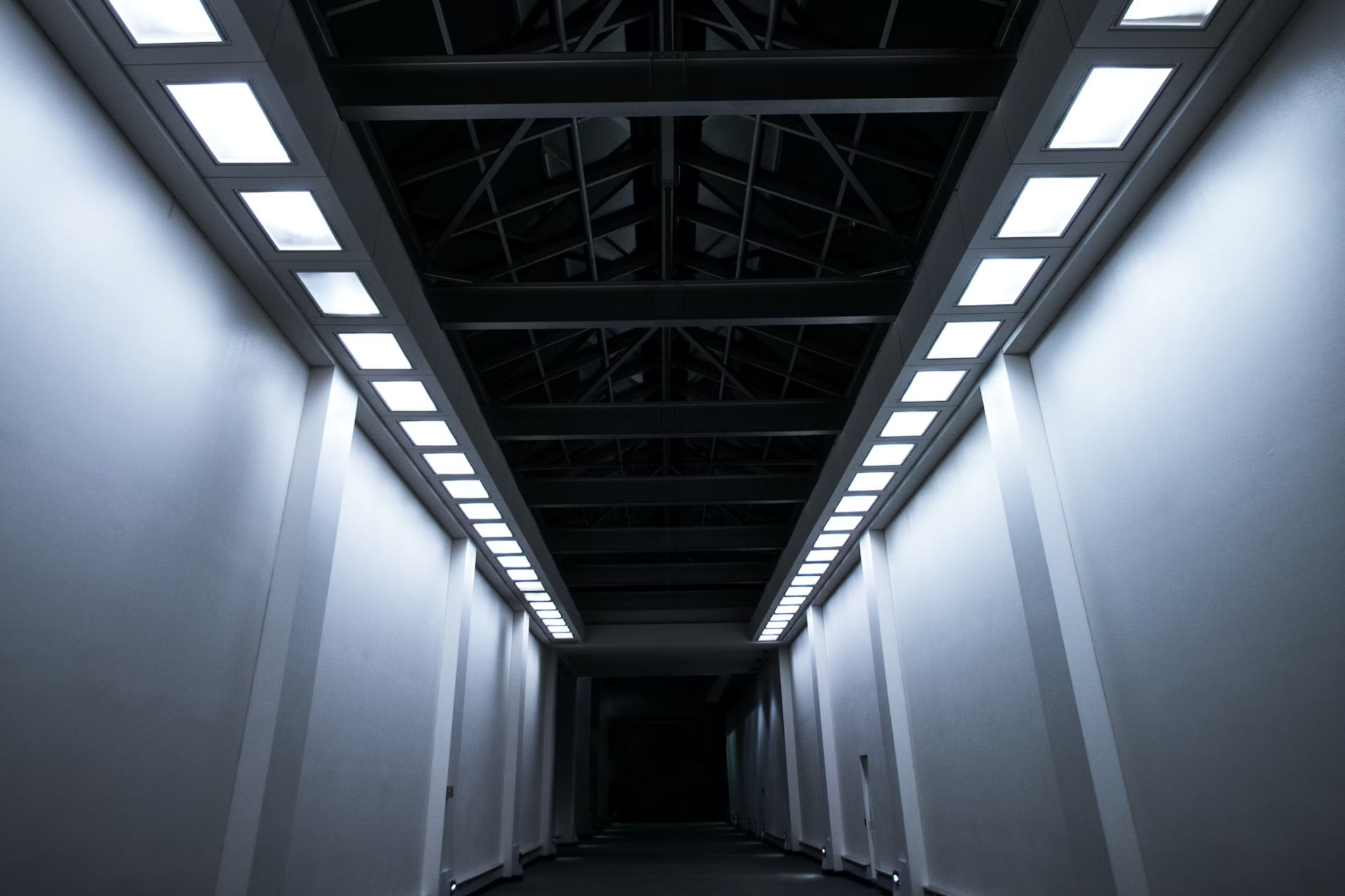
Nits, Lux, Lumen, Candela - calculating with light and lighting
Luminous flux, luminous intensity, luminance and co. - when is which quantity used and how can they be compared?